Microbiologists work with NASA to observe E. coli in space to find more efficient biofuel
by Matt Jardin |
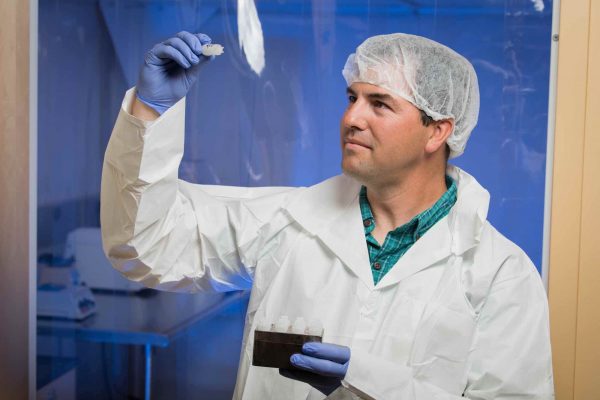
Dr. Brandon Briggs is an assistant professor of biological sciences at UAA who has recently worked with NASA to send genetically engineered strains of E. coli to the International Space Station. By studying E. coli under the microgravity conditions of space, Briggs is hoping to find a way to produce the biofuel isobutene more efficiently, without the use of oil. (Photo by James Evans / University of Alaska Anchorage)
For many, collaborating with NASA is a lifelong dream that takes years of hard work. For UAA assistant professor of biological sciences Dr. Brandon Briggs, the opportunity came as a welcome surprise.
For the past three years, Briggs has worked as principal investigator on a project researching a way to produce the biofuel known as isobutene more efficiently.
If you're unfamiliar with the chemical compound isobutene, then you're in good company. Even the word processor used to compose this story prompted, "Did you mean isobutane?"
Isobutene is a gas that serves as a key component in a variety of everyday products. Rubbers, plastics, detergents, lubricants and gasoline additives all rely on isobutene.
Currently, the only method to develop isobutene is a process known as steam cracking, which takes crude oil and subjects it to high pressure and high temperatures. Steam cracking is very energy intensive and is a significant contributor of carbon dioxide and methane emissions.
So naturally, the door is open for someone to discover a better way.
Upon first glance, the pairing might seem odd. The development of compounds like isobutene is traditionally a chemistry affair, and Briggs' experience is firmly rooted in the biological sciences. But, as the old axiom goes, sometimes you have to think outside the box.
Briggs proposes utilizing microscopic organisms, or microbes to produce isobutene more efficiently. Microbes are able to consume and convert a wide variety of compounds, and harnessing that ability is key to making isobutene without relying on steam cracking.
"That's one of the reasons I love microbiology. Microbes are incredibly diverse," Briggs explains. "As an example, humans are only able to breathe and get energy from oxygen. Microbes can do it from oxygen or they can do it from iron - they can breathe solid iron! There's a whole suite of other compounds they can get energy from. We can actually take these microbes and have them produce isobutene without high temperature and high pressure, and it's not going to come from oil."
Instead of oil, Briggs aims to use wastewater as the fuel source for microbes to convert into isobutene. The overabundance of wastewater continues to be a global concern, especially in rural communities across Alaska where refuse piles up until it can be sent away via barge.
The next step was to choose the microbe best equipped to break down wastewater. Briggs chose E. coli. While normally known to elicit fears of food poisoning, Briggs reassures that E. coli is the right microbe for the job.
"There are many different types of E. coli," he describes. "The type you hear about in the news is a particular strain that is hemorrhagic, so you bleed from it. The E. coli we're using is a different strain that is actually found in everybody's digestive system, so it's a normal part of our own flora. It actually helps us digest food, it's good at breaking down lipids in waste and it's one of the first ones we were able to easily cultivate in the lab. We know a lot more about E. coli than some other types of microbes, so it makes it a little easier to work with."
Despite having found a plentiful fuel source and the biological means to generate isobutene, Briggs' method isn't yet economically viable, stating, "We need to produce more isobutene faster to be able to compete with oil or at least be an alternative."
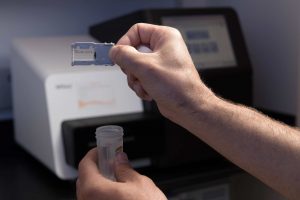
By analyzing and comparing E. coli strains under various conditions and stressors, Briggs aims to engineer the ideal strain of E. coli capable of producing large quantities of isobutene. The results of each observation appear as a genetic sequence such as this one. (Photo by James Evans / University of Alaska Anchorage)
To do that, Briggs and graduate researcher James Wilson have been working to genetically engineer a strain of E. coli capable of outputting more significant amounts of isobutene.
"If you eat a jelly doughnut, we could trace the carbon through the different pathways and see how it produces energy for us or use it to build our bodies. If we do that for E. coli, same with ours, we can see that it spits up into multiple pathways for multiple purposes. What we want is for all of it to go into isobutene," explains Briggs. "We have these competing pathways, but we don't know what they are yet. But by putting it under different conditions, we know they're going to be a little bit faster or a little more streamlined. By comparing the growth across different conditions, we can look at what those competing pathways are. Then we can go back and genetically engineer again. We can take out those competing pathways so that all the carbon from that jelly doughnut can go to producing more isobutene."
Wilson is responsible for developing the methodology to genetically engineer this ideal strain of E. coli and measure its isobutene output. Using a method known as CRISPR - abbreviated from Clustered Regularly Interspaced Short Palindromic Repeats - he is able to alter the DNA in a strain of E. coli to possess different enzymes that hopefully make it better at producing isobutene. After observing the new E. coli strain under a different condition, he interprets that data using a genetic sequencer and makes the necessary alterations in the next strain of E. coli.
"The first year, I was mainly doing the genetic engineering to put plasmid genes in the central chromosome of E. coli to get rid of the need to use antibiotics," recalls Wilson. "The reason we were doing that is because if you want to have a long-term biofuel production strain, you don't want to have to constantly be adding antibiotics to grow it and you don't want to be pumping that out into the environment. So we found that workaround by using this CRISPR system instead. Since then, we've got that engineered strain up and going. Now I'm looking at a couple of other things, like increasing production efficiency."
[embed]https://www.youtube.com/watch?v=W80DJqJk8yw[/embed]
Since the project began, Briggs and Wilson have studied their genetically engineered E. coli strains under a variety of conditions and stressors, each conveniently recreated in their lab. During which time, Briggs' hardware partner and manufacturer of the incubators that make it possible to grow the E. coli, Space Technology and Advanced Research Systems (STaARS), offered to connect them with NASA. The idea was to study E. coli under microgravity conditions by sending samples up to the International Space Station (ISS) where STaARS also has an incubator.
The choice was easy, both for Briggs who had the chance to add a new condition to the study - and in space of all places - and for NASA who wanted to fast-track the project. In just two months, down from the normal 18-month lead time NASA provides, Briggs was able to reoutfit the vials to withstand conditions on the ISS, complete preliminary studies and fill out a ton of paperwork.
This past May, NASA sent up Briggs and Wilson's E. coli samples to the ISS orbiting 240 miles above Earth. For this experiment, Briggs organized for the astronauts to unfreeze the samples at different intervals and place them in an incubator to record its growth. The samples are expected to remain in the ISS until the end of the year before being refrozen, sent back down to Earth and shipped back to UAA for the team to once again undergo the process of analyzing the data and reengineering a new strain.
"We'll have to see what the data says. Basically we repeat. We'll go back to genetic engineering. We'll go back and try to remove some of these competing pathways or increase production of something that will help it produce more isobutene," says Briggs. "It's a reiterative project where we'll engineer something, see what happens, and keep going until we can get to those high enough levels of isobutene production."
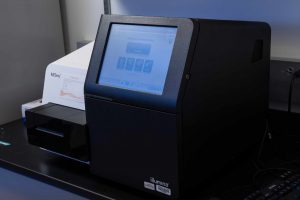
After observing E. coli in a new condition, the results are fed into a genetic sequencer where they are compared with the performance of other E. coli strains under different conditions. (Photo by James Evans / University of Alaska Anchorage)
Wilson adds, "With that number of samples, it's probably going to be about four or five weeks to get everything ready and sequenced. Then it will probably be about another month or so of computer time to dive through everything and run the code to figure out what works."
Until the E. coli samples are back planetside, NASA made sure Briggs and Wilson had something to alleviate the wait.
Wilson was given the opportunity to fly down to Virginia to watch the shuttle launch and meet the director of NASA.
"Since getting into science, I've been interested in working with space and sending things out, things along that nature. To finally have that chance has been wonderful and I'm really grateful to Brandon for putting in years of work before I showed up to be able to do this," says Wilson.
Although he was unable to attend the launch, Briggs still managed to get a cool souvenir.
"There was a little snafu and I got a panicked phone call at four in the morning asking, 'What do we do?!'" Briggs recalls. "The astronaut called back down to Houston and recorded a very nice message for me, 'You just got a phone call from space - Collect!'
Written by Matt Jardin, UAA Office of University Advancement
